Medical CT
Computed tomography (CT) combines a series of X-ray images taken from different angles around the body and uses computer processing to create cross-sectional images (slices) of the body’s internal bones, blood vessels and soft tissues. CT scans provide more detailed information than ordinary X-rays.
A CT scan has many uses, but it is especially good for quickly examining people who may have suffered internal injuries from a car accident or other type of trauma. CT scans can show almost any part of the body and are used to diagnose illness or injury, as well as to plan medical treatment, surgery or radiation.
The workflow of signal detection and processing in CT is shown in a simplified form in Fig. 1. In the X-ray CT process chain, the detection step is carried out by means of physical detectors, which capture the attenuated X-ray beam and convert it into electrical signals, which are subsequently converted into binary coded information. As depicted in Fig. 1, after several processing steps, the results are available for final visualisation and interpretation on a computer.
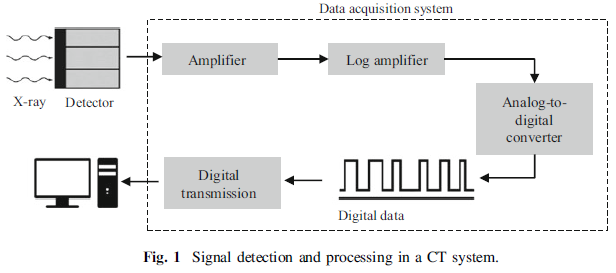
In conventional attenuation-based CT, the X-rays are attenuated due to absorption or scattering during their travel through the material. The attenuation is then measured by capturing the attenuated X-rays using an X-ray detector. Over the years, there has been a considerable improvement in the detection technology. The detector captures the X-ray beam and converts it into electrical signals. This conversion of X-ray energy to electrical signals is primarily based on two principles: gas ionisation detectors and scintillation (solid-state) detectors. A schematic representation of both detection principles is shown in Fig. 2. Gas ionisation detectors convert X-ray energy directly into electrical energy, whereas scintillation detectors convert X-rays into visible light and then the light is converted into electrical energy.
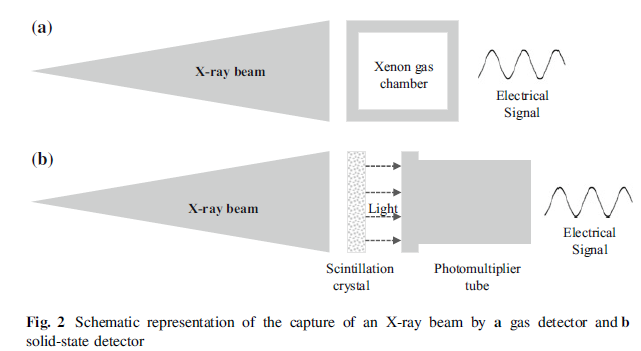
When the X-rays strike the scintillator crystals, they are converted into long-wave radiation (visible light) within the scintillation media. The light is then directed to an electronic light sensor such as a photomultiplier tube. The photomultiplier absorbs the light emitted by the scintillator and ejects electrons via the photoelectric effect. Electrons are released when the light strikes the photocathode and then the electrons cascade through a series of dynodes, maintained at different potentials, to result in an output signal.
The choice of scintillator material is critical and depends on the desired quantum efficiency for the X-ray-to-light conversion, and on the time constant for the conversion process, which determines the “afterglow” of the detector (i.e. the persistence of the image even after turning off the radiation, as described in the following section). Modern sub-second scanners require a very small time constant (or very fast fluorescence decay). Typical materials for scintillators are sodium iodide doped with thallium caesium iodide cadmium tungstate (CdWO4), zinc sulphide (ZnS), naphthalene, anthracene and other compounds based on rare earth elements, or gadolinium oxysulfide (Gd2O2S).
Scintillation crystals used in medical CT
Ce:YAG
Wavelength (Max. Emission) (nm) | 550 |
Decay time (ns) | 70 |
Light yield (Photons/kev) | 35 |
Refractive index | 1.82@550nm |
Radiation length (cm) | 3.5 |
In terms of maximum capture rate, YAP (Ce) shows better performance than YAG (Ce). However, the YAG (Ce) crystal was chosen because its emission spectrum is comparable to the sensitivity of the photodiode. The calorimeter must operate in a particle accelerator processing chamber where the magnetic field is not negligible, so the use of photodiodes in the readout system is preferable to the standard photomultiplier tubes required for YAP (Ce) crystals. In addition, the results using a photodiode calorimeter are more compact and allow for a reduction in the size of the overall pCT device.
Ce:YAG(Y3Al5O12) | Density (g/cm3) | Max. emission wavelength (nm) | Decay constant (ns) |
Ref[1] | / | 670 | 100μs |
Ref[2] | / | 670 | 75 |
Ref[3] | 4.55 | 550 | 88 |
References
[1] Design and characterisation of a YAG(Ce) calorimeter for proton Computed Tomography application
[2] Characterization of a Silicon Strip Detector and a YAG:Ce Calorimeter for a Proton Computed Radiography Apparatus
[3] A High Speed Functional MicroCT Detector For Small Animal Studies
Ce:YAP
Wavelength (Max. Emission) (nm) | 370 |
Decay time (ns) | 28 |
Light yield (Photons/kev) | 25 |
Light output relative to Nal (TI) (%) | 60-70 |
Refractive index | 1.95@370nm |
YAP (Ce) is commonly used as a photon-loaded scintillator in medical devices and environmental monitoring detectors due to its high light yield, good resolution, hardness and non-hygroscopicity. Unfortunately, such crystals have a slow decay time in flicker light. This limits their high frequency applications. In this case, it has been investigated how such crystals can be used as a calorimeter for protons to enter particles at higher speeds, as in proton computed tomography.
Ce:YAP(YAlO3) | Density (g/cm3) | Max. emission wavelength (nm) | Decay constant (ns) |
Ref[1] | 5.37 | 370 | 25 |
Ref[2] | / | 370 | 28 |
Ref[3] | / | 370 | 10 |
References
[1] YAP(Ce) crystal characterization with proton beam up to 60 MeV
[2] Mcps-range photon-counting X-ray computed tomography system utilizing an oscillating linear-YAP(Ce) photon detector
[3] Dark-count-less photon-counting X-ray computed tomography system using a YAP-MPPC detecto
CsI
Wavelength (Max. Emission) (nm) | 410 |
Decay time (ns) | 40 |
Light yield (Photons/kev) | 25 |
Light output relative to Nal (TI) (%) | 75 |
Refractive index | 1.82@410nm |
Compared with the traditional aS1000 model consisting of 0.4 mm thick gadolinium oxide sulfur scintillation, the CsI detector has an 8 mm thick CsI crystal with higher X-ray absorption efficiency.
CsI | Density (g/cm3) | Max. emission wavelength (nm) | Decay constant (ns) |
Ref[1] | 4.51 | 540 | 680 |
References
[1] A High Speed Functional MicroCT Detector For Small Animal Studies
Ce:LYSO
Wavelength (Max. Emission) (nm) | 410 |
Decay time (ns) | 40 |
Light yield (Photons/kev) | 25 |
Light output relative to Nal (TI) (%) | 75 |
Radiation length (cm) | 1.82@410nm |
Ce:LYSO is a single crystal non-hygroscopic scintillator with high density, high light yield and short decay time, which has been successfully used in medical CT imagers.
Ce:LYSO(LuYSiO5) | Density (g/cm3) | Max. emission wavelength (nm) | Decay constant (ns) |
Ref[1] | 7.1 | 420 | 48 |
Ref[2] | 7.1 | 420 | 40 |
Ref[3] | 7 | 420 | / |
References
[1] YAP(Ce) crystal characterization with proton beam up to 60 MeV
[2] Luminescence Emission Properties of (Lu; Y)2SiO5:Ce (LYSO:Ce) and (Lu; Y)AlO3:Ce (LuYAP:Ce) Single Crystal Scintillators Under Medical Imaging Conditions
[3] A comparative study of the luminescence properties of LYSO:Ce, LSO:Ce, GSO:Ce and BGO single crystal scintillators for use in medical X-ray imaging
Ce:LuAG
Wavelength (Max. Emission) (nm) | 535 |
Wavelength range (nm) | 475-800 |
Decay time (ns) | 70 |
Light yield (Photons/kev) | 25 |
Refractive index | 1.84@633nm |
High detection efficiency can be achieved by using materials with high density and high Ordinal Numbers. The 5D to 4F fast luminescence of Ce3+ is usually utilized, with a unique 20-60 ns decay time to provide efficient emission at room temperature. Lu3Al5O12 doped with Ce is a well-known scintillator with ideal physical and scintillation properties.
References
[1] Crystal growth and characterization of LuAG:Ce:Tb scintillator
[2] Epitaxial growth of LuAG:Ce and LuAG:Ce,Pr films and their scintillation properties
BGO
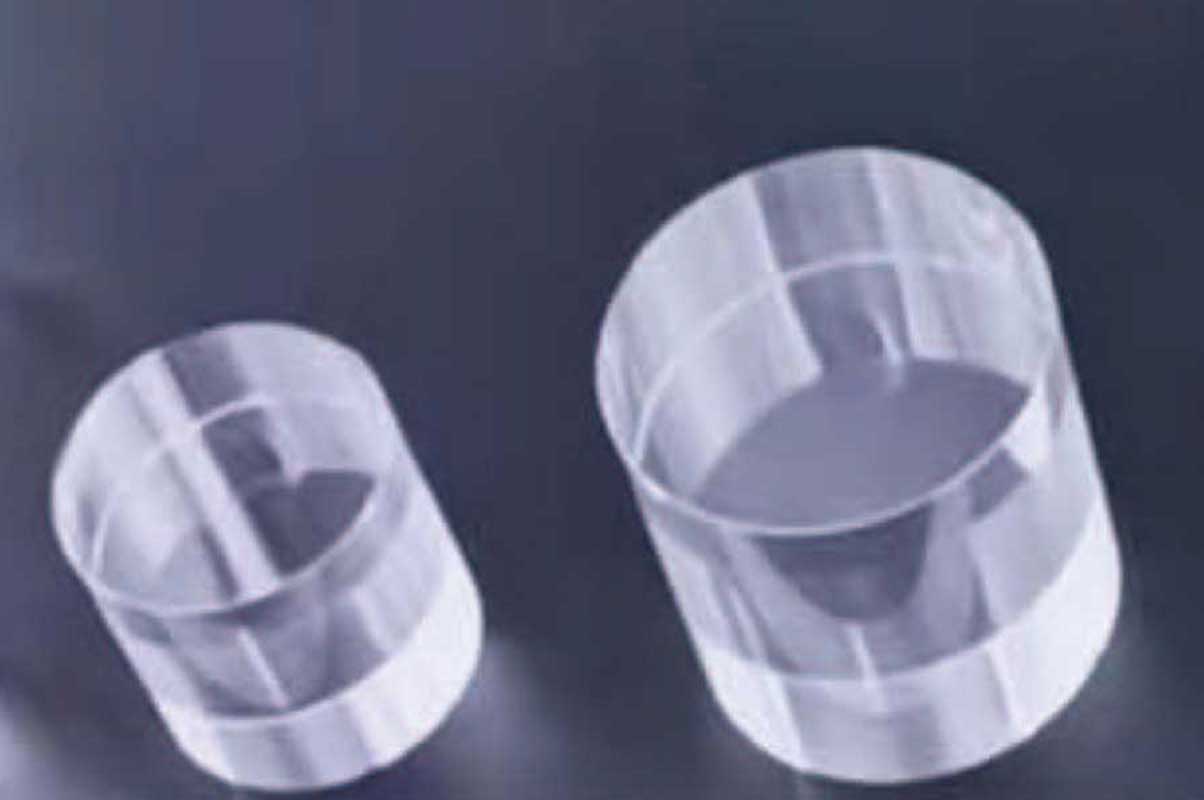
Wavelength (Max. Emission) (nm) | 480 |
Decay time (ns) | 300 |
Light yield (Photons/kev) | 8-10 |
Refractive index | 2.15 |
Energy resolution (%) | 12 |
Bismuth germanate (BGO; Bi4Ge3O12) has high X-ray cutoff power, but its relatively low scintillation efficiency (8-16% of NaI (TI)) and long scintillation decay time (300ns) require effective optical coupling with photomultiplier tubes to obtain good temporal resolution and reasonable energy resolution.
References
[1] A QUAD BGO DETECTOR AND ITS TIMING AND POSITIONING DISCRIMINATION FOR POSITRON COMPUTED TOMOGRAPHY
[2] Plastic scintillation detectors for high resolution emission computed tomography.
[3] YAP(Ce) crystal characterization with proton beam up to 60 MeV
CdWO4
Wavelength (Max. Emission) (nm) | 490 |
Decay time (ns) | 14000 |
Light yield (Photons/kev) | 12-15 |
Light output relative to Nal (TI) (%) | 50 |
Refractive index | 2.2-2.3 |
Cadmium tungstate (CWO) single crystals are widely used in X-ray computed tomography and endoscopy, spectroscopy and radiometry equipment for the manufacture of small detectors with photodiodes or photoamplifiers and multielement detection components for computed tomography (CT) scans.
CdWO4 | Density (g/cm3) | Max. emission wavelength (nm) | Decay constant (ns) |
Ref[1] | 7.9 | / | / |
Ref[2] | / | 470-580 | >1000 |
Ref[3] | / | 480-500 | / |
References
[1] A bench-top megavoltage fan-beam CT using CdWO4-photodiode detectors. I. System description and detector characterization
[2] Production ofthe high-quality CdWO4 single crystals for application in CT and radiometric monitoring
[3] MONOLITHICALLY INTEGRATED X-RAY DETECTOR ARRAYS FOR COMPUTED TOMOGRAPHY
LaBr3
Wavelength (Max. Emission) (nm) | 380 |
Decay time (us) | 25 |
Light yield (Photons/kev) | 63 |
Radiation length (cm) | 1.881 |
Reflection loss/surface (%) | 6.3 |
LaBr3 (Ce) – lanthanum bromide – is a salt compound of lanthanum and bromine, which is one of the new generation of γ-radiation detectors based on inorganic scintillators. LaBr3 (Ce) scintillators have a fast decay time of light output and excellent energy resolution performance.
LaBr3 | Density (g/cm3) | Light output [Nal (Tl) (%)] | Decay constant (ns) |
Ref[1] | 5.08 | 160 | 26 |
References
[1]A Study on Determination of an Optimized Detector for Single Photon Emission Computed Tomography
GOS
Wavelength (Max. Emission) (nm) | 510 |
Decay time (us) | 5.5 |
Relative light output (%) | 80 |
Afterglow (%) | <0.01 |
Luminescence intensity (keV) | 27.5 |
References
[1]Improvements in the X-ray Characteristics of Gd2O2S:Pr Ceramic Scintillators